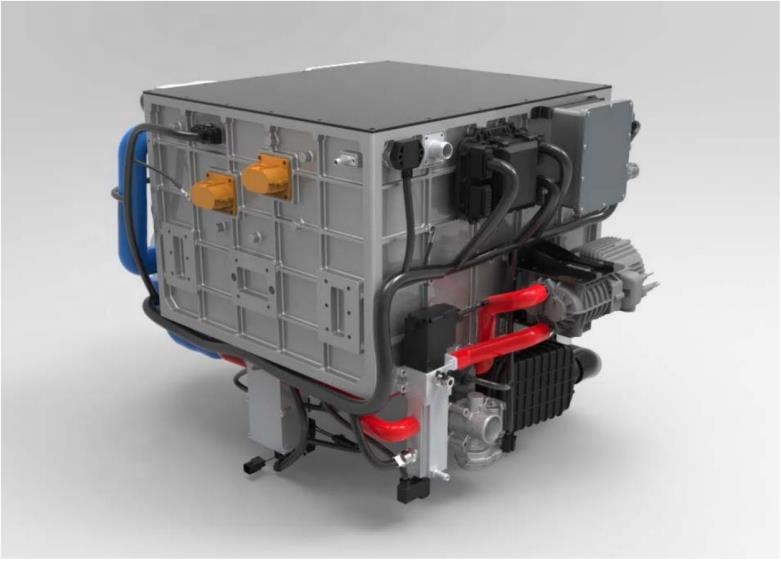
Fuel cell stacks represent a groundbreaking innovation in clean energy technology. By converting hydrogen into electricity through an electrochemical process, they power electric vehicles with remarkable efficiency. Unlike traditional engines, this system produces no harmful emissions, releasing only water vapor as a byproduct. Countries like Japan, Germany, and the United States actively promote fuel cell vehicles to combat greenhouse gas emissions. With advancements in materials and designs, fuel cell systems now achieve efficiency ratings of up to 60%, surpassing internal combustion engines. This transformative technology is paving the way for a sustainable future in transportation.
要点
- Fuel cell stacks convert hydrogen into electricity with up to 60% efficiency, making them a highly effective energy source for electric vehicles.
- Unlike traditional engines, fuel cell stacks produce zero harmful emissions, releasing only water vapor, which contributes to environmental sustainability.
- FCEVs can be refueled in under five minutes, offering a significant advantage over battery-powered vehicles, which require longer charging times.
- The modular design of fuel cell stacks allows for scalability, enabling customization for various vehicle types, from passenger cars to heavy-duty trucks.
- Fuel cell technology addresses range anxiety, with many FCEVs capable of traveling over 300 miles on a single hydrogen fill-up.
- Regular maintenance and monitoring are essential for optimizing the performance and longevity of fuel cell stacks, ensuring their reliability in real-world applications.
- Innovations by companies like Ningbo VET Energy Technology Co. are driving advancements in fuel cell technology, enhancing efficiency and durability for broader adoption.
Understanding the Role of Fuel Cell Stacks in Electric Vehicles
What is a Fuel Cell Stack?
A fuel cell stack serves as the central component of a fuel cell electric vehicle (FCEV). It consists of multiple individual fuel cells arranged in series or parallel to generate the required power output. Each fuel cell within the stack contains two electrodes—an anode and a cathode—separated by an electrolyte. This modular design allows the stack to produce higher voltage and current, meeting the energy demands of electric vehicles.
The fuel cell stack operates on the principle of “cold combustion,” where hydrogen and oxygen react chemically to produce electricity. Unlike traditional combustion engines, this process emits no pollutants. The only byproducts are water, electricity, and heat, making it an environmentally friendly solution for transportation.
How Fuel Cell Stacks Generate Electricity
Fuel cell stacks generate electricity through an electrochemical reaction. Hydrogen, stored in high-pressure tanks, enters the anode side of the fuel cell. Simultaneously, oxygen from the air flows into the cathode side. At the anode, hydrogen molecules split into protons and electrons. The protons pass through the electrolyte, while the electrons travel through an external circuit, creating an electric current.
At the cathode, the protons, electrons, and oxygen combine to form water. This reaction releases heat, which can be utilized for other vehicle functions. By connecting multiple fuel cells in a stack, the system achieves the necessary power output to drive the electric motor of the vehicle. This process ensures high energy efficiency, with some fuel cell stacks achieving efficiency ratings of up to 60%.
Importance of Fuel Cell Stacks in FCEVs
Fuel cell stacks play a pivotal role in the functionality of FCEVs. They provide a clean and efficient energy source, enabling vehicles to operate without emitting harmful pollutants. This technology addresses the growing demand for sustainable transportation solutions, especially in regions prioritizing the reduction of greenhouse gas emissions.
The advantages of fuel cell stacks extend beyond environmental benefits. They offer faster refueling times compared to battery-powered electric vehicles, making them suitable for long-distance travel and heavy-duty applications. Additionally, their modular design ensures scalability, allowing manufacturers to customize power outputs for various vehicle types, from passenger cars to industrial trucks.
By integrating fuel cell stacks, FCEVs combine the benefits of hydrogen fuel and electric propulsion. This synergy enhances energy efficiency, driving range, and overall performance, positioning fuel cell technology as a key player in the future of sustainable mobility.
How Fuel Cell Stacks Work
The Electrochemical Process
The operation of a fuel cell stack relies on an electrochemical process that converts hydrogen and oxygen into electricity. This process begins when hydrogen gas enters the anode side of the fuel cell. At the anode, a catalyst splits hydrogen molecules into protons and electrons. The protons pass through the electrolyte, while the electrons travel through an external circuit, generating an electric current. This current powers the electric motor of the vehicle.
On the cathode side, oxygen from the air reacts with the protons and electrons to form water. This reaction also produces heat, which can be utilized for auxiliary functions within the vehicle. The entire process occurs without combustion, ensuring zero harmful emissions. The electrochemical process is highly efficient, with some fuel cell stacks achieving energy conversion rates of up to 60%.
Components of a Fuel Cell Stack
A fuel cell stack consists of several key components that work together to generate electricity:
- Anode: The anode serves as the entry point for hydrogen gas. It contains a catalyst that facilitates the splitting of hydrogen molecules into protons and electrons.
- Cathode: The cathode is where oxygen reacts with protons and electrons to produce water and heat.
- Electrolyte: The electrolyte allows only protons to pass through, ensuring that electrons travel through the external circuit to generate electricity.
- Bipolar Plates: These plates distribute gases evenly across the electrodes and conduct electricity between individual fuel cells.
- Membrane Electrode Assembly (MEA): The MEA combines the anode, cathode, and electrolyte into a single unit, optimizing the efficiency of the electrochemical reaction.
Each fuel cell in the stack operates independently, but when connected in series or parallel, they collectively produce the voltage and current required to power an electric vehicle.
Role of Hydrogen and Oxygen in Energy Generation
Hydrogen and oxygen play crucial roles in the energy generation process of a fuel cell stack. Hydrogen acts as the primary fuel source, while oxygen serves as the oxidizing agent. The interaction between these two elements drives the electrochemical reaction that produces electricity.
Hydrogen is stored in high-pressure tanks within the vehicle. When released into the fuel cell stack, it undergoes a catalytic reaction at the anode. This reaction separates hydrogen into protons and electrons, initiating the flow of electricity. Oxygen, drawn from the surrounding air, enters the cathode side of the fuel cell. It combines with the protons and electrons to form water, which exits the system as a harmless byproduct.
The use of hydrogen and oxygen ensures a clean and efficient energy generation process. Unlike traditional combustion engines, fuel cell stacks emit only water vapor and warm air, making them an environmentally friendly alternative for powering electric vehicles.
Advantages of Fuel Cell Stacks in Electric Vehicles
Faster Refueling Compared to Batteries
Fuel cell vehicles (FCEVs) excel in refueling speed, offering a significant advantage over battery-powered electric vehicles. Hydrogen fuel tanks in FCEVs can be fully refueled in less than four minutes. This process mirrors the convenience of refueling traditional gasoline vehicles, making it ideal for drivers who prioritize time efficiency. In contrast, battery electric vehicles often require 20 minutes to an hour for an 80% charge, even with fast-charging technology. For heavy-duty commercial vehicles or fleets that operate continuously, the rapid refueling capability of fuel cell stacks ensures minimal downtime and maximized productivity.
Longer Driving Range and High Efficiency
Fuel cell stacks provide FCEVs with an extended driving range, addressing one of the primary concerns associated with electric vehicles—range anxiety. On a single hydrogen fill-up, many FCEVs can travel over 300 miles, surpassing the range of most battery-powered electric vehicles. This makes them particularly suitable for long-distance travel and applications requiring consistent performance over extended periods. Additionally, the high energy conversion efficiency of fuel cell stacks, which can reach up to 60%, ensures that the energy derived from hydrogen is utilized effectively. This efficiency, combined with the longer range, positions FCEVs as a practical solution for both personal and commercial use.
Zero Emissions and Environmental Benefits
Fuel cell stacks contribute significantly to environmental sustainability by producing zero harmful emissions. The only byproducts of the electrochemical reaction within the stack are water vapor and warm air. This clean energy generation process helps reduce greenhouse gas emissions, aligning with global efforts to combat climate change. Unlike internal combustion engines, which release pollutants into the atmosphere, FCEVs powered by fuel cell stacks offer a cleaner alternative for transportation. Their environmental benefits make them an attractive option for countries and organizations aiming to achieve carbon neutrality.
Efficiency of Fuel Cell Stacks
Energy Conversion Efficiency
Fuel cell stacks demonstrate exceptional energy conversion efficiency, setting them apart from traditional energy systems. By directly converting chemical energy from hydrogen into electrical energy, these stacks achieve efficiency ratings of 40-60%. This range significantly surpasses the efficiency of internal combustion engines, which typically operate at 20-35%. The absence of mechanical energy conversion processes, such as those found in combustion engines, allows fuel cell stacks to minimize energy losses and maximize output.
In real-world applications, fuel cells installed in the United States have achieved an electrical efficiency of 46%, outperforming coal plants (38%) and gas turbines (40-57%). This high efficiency not only reduces energy waste but also enhances the overall performance of fuel cell electric vehicles (FCEVs). The ability to convert energy with such precision makes fuel cell stacks a cornerstone of sustainable transportation technology.
Comparison with Internal Combustion Engines
Fuel cell stacks offer a clear advantage over internal combustion engines in terms of efficiency and environmental impact. While internal combustion engines rely on burning fossil fuels, which results in significant energy loss through heat, fuel cell stacks utilize an electrochemical process that directly generates electricity. This process enables fuel cell stacks to achieve efficiency levels of up to 60%, more than twice the efficiency of many combustion engines, which average around 27%.
The environmental benefits further highlight the superiority of fuel cell stacks. Internal combustion engines emit harmful pollutants, including carbon dioxide and nitrogen oxides, contributing to air pollution and climate change. In contrast, fuel cell stacks produce only water vapor and warm air as byproducts, making them a cleaner and more sustainable alternative. These advantages position fuel cell stacks as a transformative technology in the transition to greener transportation solutions.
Contributions by Ningbo VET Energy Technology Co.
Ningbo VET Energy Technology Co. plays a pivotal role in advancing the efficiency and reliability of fuel cell stacks. Through continuous research and development, the company has introduced innovative designs and materials that enhance the performance of fuel cell systems. Their contributions include optimizing the membrane electrode assembly (MEA) and improving the durability of key components, ensuring long-term efficiency and stability.
The company’s commitment to innovation extends to the integration of cutting-edge cooling mechanisms and temperature control systems. These advancements help maintain optimal operating conditions for fuel cell stacks, further boosting their energy conversion efficiency. By addressing technical challenges and pushing the boundaries of fuel cell technology, Ningbo VET Energy Technology Co. solidifies its position as a leader in the field, driving the adoption of fuel cell stacks in electric vehicles worldwide.
Operational Considerations for Fuel Cell Stacks
Temperature Control and Cooling Mechanisms
Fuel cell stacks operate efficiently within a specific temperature range. Excessive heat can degrade components, while low temperatures may hinder the electrochemical reaction. Effective temperature control ensures optimal performance and longevity. Manufacturers integrate advanced cooling mechanisms to regulate heat generated during operation.
A common cooling method involves liquid cooling systems. These systems circulate coolant through channels in the fuel cell stack, dissipating heat effectively. Bipolar plates, a key component of the stack, often include integrated cooling channels to enhance thermal management. Air cooling, though less efficient, is sometimes used in smaller systems due to its simplicity.
Research highlights the importance of maintaining consistent thermal conditions. Studies on performance changes under varying environments, such as those published in PMC, emphasize that temperature fluctuations can impact efficiency and durability. For instance, uneven cooling may lead to “hot spots,” which accelerate material degradation. By addressing these challenges, manufacturers ensure that fuel cell stacks deliver reliable and consistent energy output.
Durability and Longevity of Fuel Cell Stacks
Durability remains a critical factor in the widespread adoption of fuel cell technology. Fuel cell stacks must withstand thousands of operational cycles without significant performance loss. Key factors influencing durability include material quality, operating conditions, and maintenance practices.
The Nature journal underscores the need for durability test cycles at the system level. These tests simulate real-world conditions, such as load variations and start-stop cycles, to evaluate long-term performance. For example, proton exchange membrane fuel cells (PEMFCs) experience wear due to repeated cycling, which can affect their efficiency over time. Advanced materials, such as corrosion-resistant catalysts and reinforced membranes, help mitigate these effects.
Manufacturers also focus on improving the structural integrity of components. Bipolar plates, for instance, are designed to resist mechanical stress and chemical corrosion. By enhancing durability, fuel cell stacks achieve longer lifespans, reducing replacement costs and increasing their appeal for commercial applications.
Maintenance Requirements for Optimal Performance
Regular maintenance plays a vital role in ensuring the optimal performance of fuel cell stacks. Proper upkeep minimizes the risk of component failure and extends the system’s operational life. Maintenance tasks typically include inspecting seals, cleaning cooling channels, and monitoring gas flow rates.
Hydrogen purity is another critical aspect. Impurities in hydrogen fuel can damage the catalyst and reduce efficiency. Periodic checks of the hydrogen supply system prevent contamination and ensure consistent performance. Additionally, monitoring software integrated into modern fuel cell systems provides real-time data on stack health, enabling proactive maintenance.
Studies on performance changes, such as those documented in PMC, reveal that external factors like fan power and flow fields influence stack efficiency. Regular adjustments to these parameters optimize energy output and prevent unnecessary wear. By adhering to a structured maintenance schedule, operators can maximize the reliability and efficiency of fuel cell stacks, ensuring their long-term viability in electric vehicles.
Challenges and Innovations in Fuel Cell Stack Technology
Current Challenges in Hydrogen Production and Storage
Hydrogen production and storage present significant challenges for the widespread adoption of fuel cell technology. Producing hydrogen often relies on energy-intensive methods, such as steam methane reforming, which emits carbon dioxide. Although renewable energy sources like solar and wind can generate green hydrogen through electrolysis, the process remains costly and less efficient. Scaling up these methods to meet global energy demands requires substantial investment and technological advancements.
Storing hydrogen also poses difficulties due to its low density. High-pressure tanks or cryogenic systems are necessary to store hydrogen in a compact form, but these solutions increase costs and complexity. Leakage risks further complicate storage, as hydrogen molecules are small and can escape through tiny gaps. Addressing these issues is essential to ensure the safe and efficient use of hydrogen in fuel cell stacks.
Efforts to overcome these challenges have gained momentum. For example, NASA’s early involvement in hydrogen fuel cells demonstrated their potential as a viable commercial resource. This paved the way for ongoing research into cost-effective production methods and advanced storage technologies. By improving these aspects, hydrogen can become a more accessible and sustainable energy source for fuel cell electric vehicles (FCEVs).
Infrastructure Development for Hydrogen Refueling
The lack of a robust hydrogen refueling infrastructure remains a major barrier to the adoption of FCEVs. Unlike gasoline stations, hydrogen refueling stations are scarce, limiting the practicality of fuel cell vehicles for everyday use. Building a comprehensive network of refueling stations requires significant investment and coordination among governments, private companies, and energy providers.
Countries like Japan and Germany have taken proactive steps to address this issue. Japan has established a national strategy to expand its hydrogen refueling network, aiming to support the growing number of FCEVs on its roads. Similarly, Germany has partnered with private firms to develop a network of hydrogen stations, ensuring accessibility for both passenger cars and commercial vehicles.
Hydrogen-powered utility vehicles, such as Renault’s Kangoo Z.E Hydrogen and Master Z.E Hydrogen, highlight the importance of infrastructure development. These vehicles offer practical solutions for businesses, but their success depends on the availability of refueling stations. Expanding infrastructure will not only support existing FCEVs but also encourage further innovation in hydrogen-powered transportation.
Future Innovations by Ningbo VET Energy Technology Co.
Ningbo VET Energy Technology Co. continues to lead advancements in fuel cell stack technology. The company focuses on enhancing the efficiency, durability, and scalability of its products to meet the evolving demands of the transportation industry. By investing in research and development, Ningbo VET Energy Technology Co. aims to address current limitations and unlock new possibilities for fuel cell applications.
One area of innovation involves integrating advanced materials into fuel cell stacks. These materials improve the performance of key components, such as the membrane electrode assembly (MEA) and bipolar plates. Enhanced durability ensures that fuel cell stacks can withstand rigorous operating conditions, extending their lifespan and reducing maintenance costs.
The company also explores cutting-edge cooling mechanisms to optimize thermal management. Maintaining consistent operating temperatures is crucial for maximizing energy efficiency and preventing component degradation. By refining these systems, Ningbo VET Energy Technology Co. ensures reliable performance across various vehicle types, from passenger cars to heavy-duty trucks.
Looking ahead, the company envisions a future where fuel cell technology plays a central role in achieving carbon neutrality. Its commitment to innovation aligns with global efforts to transition toward sustainable energy solutions. Through continuous advancements, Ningbo VET Energy Technology Co. solidifies its position as a pioneer in the field, driving the adoption of fuel cell stacks worldwide.
Comparing Fuel Cell Stacks to Other EV Technologies
Fuel Cell Stacks vs. Battery-Powered EVs
Fuel cell stacks and battery-powered electric vehicles (BEVs) represent two distinct approaches to sustainable transportation. While both technologies aim to reduce carbon emissions, their operational characteristics differ significantly.
-
Refueling and Charging Time
Fuel cell stacks excel in refueling speed. Hydrogen tanks in fuel cell electric vehicles (FCEVs) can be refueled in under five minutes, similar to traditional gasoline vehicles. In contrast, BEVs require longer charging times, ranging from 20 minutes to several hours, depending on the charger type. This makes fuel cell stacks more suitable for applications requiring minimal downtime, such as commercial fleets or long-distance travel. -
Driving Range
FCEVs powered by fuel cell stacks often achieve longer driving ranges compared to BEVs. Many FCEVs can travel over 300 miles on a single hydrogen fill-up, while most BEVs offer ranges between 200 and 300 miles. This extended range positions fuel cell stacks as a practical solution for heavy-duty vehicles and intercity transportation. -
Energy Efficiency and Emissions
Both technologies produce zero tailpipe emissions, contributing to environmental sustainability. However, fuel cell stacks offer high energy efficiency, particularly in scenarios requiring consistent power output over long distances. BEVs, on the other hand, are more efficient in urban settings with frequent stops and starts. -
Infrastructure Availability
BEVs benefit from a more established charging infrastructure, with numerous public and private charging stations available globally. In contrast, hydrogen refueling stations remain limited, posing a challenge for the widespread adoption of FCEVs. Countries like Japan and Germany are actively expanding their hydrogen networks to address this gap.
“Both BEVs and FCEVs play crucial roles in achieving global decarbonization goals. Their complementary strengths cater to different use cases, ensuring a balanced approach to sustainable mobility.”
Fuel Cell Stacks vs. Hybrid Systems
Hybrid systems combine internal combustion engines with electric motors, offering a transitional solution between traditional and fully electric vehicles. When compared to fuel cell stacks, several key differences emerge:
-
Emissions
Fuel cell stacks produce zero emissions, emitting only water vapor and warm air. Hybrid systems, while more efficient than conventional engines, still rely on fossil fuels and emit greenhouse gases. This makes fuel cell stacks a cleaner alternative for environmentally conscious consumers. -
Energy Source
FCEVs utilize hydrogen as their primary energy source, enabling renewable energy integration. Hybrid systems depend on gasoline or diesel, limiting their potential for complete decarbonization. The reliance on fossil fuels also impacts the long-term sustainability of hybrid vehicles. -
Performance and Scalability
Fuel cell stacks offer scalability by increasing the number of cells in the stack, allowing manufacturers to customize power outputs for various vehicle types. Hybrid systems, constrained by their dual-engine design, lack this flexibility. This makes fuel cell stacks more versatile for applications ranging from passenger cars to industrial trucks. -
Refueling and Maintenance
FCEVs refuel quickly, providing a seamless experience similar to traditional vehicles. Hybrid systems, while convenient, require regular maintenance for both the internal combustion engine and the electric motor. This dual maintenance need increases operational complexity and costs.
“Fuel cell stacks represent a forward-looking technology, while hybrid systems serve as a bridge to fully sustainable solutions. Their differences highlight the evolving landscape of clean energy transportation.”
Use Cases for Each Technology
Each technology—fuel cell stacks, BEVs, and hybrid systems—addresses specific transportation needs. Understanding their ideal applications helps clarify their roles in the future of mobility.
-
Fuel Cell Stacks
- Best suited for long-distance travel and heavy-duty applications.
- Ideal for buses, trucks, and industrial vehicles requiring high power and quick refueling.
- Effective in regions with developing hydrogen infrastructure, such as Japan and Germany.
-
Battery-Powered EVs
- Optimal for urban and suburban environments with established charging networks.
- Suitable for personal vehicles and short commutes.
- Preferred for areas prioritizing renewable electricity generation.
-
Hybrid Systems
- Practical for regions lacking robust EV infrastructure.
- Useful for drivers seeking improved fuel efficiency without transitioning fully to electric.
- Commonly adopted in markets where gasoline remains the dominant energy source.
“The diverse use cases of these technologies underscore the importance of a multi-faceted approach to sustainable transportation. Each plays a vital role in addressing unique challenges and opportunities within the industry.”
Real-World Applications of Fuel Cell Stacks
Passenger Cars and Public Transportation
Fuel cell stacks have revolutionized passenger cars and public transportation by providing clean and efficient energy solutions. In passenger vehicles, they enable long driving ranges and quick refueling, making them ideal for daily commutes and long-distance travel. Automakers like Toyota and Hyundai have introduced fuel cell electric vehicles (FCEVs) such as the Toyota Mirai and Hyundai Nexo, showcasing the potential of this technology in personal transportation.
Public transportation systems also benefit significantly from fuel cell stacks. Buses powered by fuel cells operate quietly and emit only water vapor, creating a cleaner and more comfortable urban environment. Cities like London and Tokyo have adopted hydrogen-powered buses to reduce air pollution and meet sustainability goals. These buses demonstrate the scalability of fuel cell stacks, as they can handle the high energy demands of public transit while maintaining zero emissions.
“Fuel cell stacks in passenger cars and public transportation represent a pivotal step toward achieving carbon-neutral mobility.”
Heavy-Duty Vehicles and Industrial Applications
Fuel cell stacks excel in heavy-duty vehicles and industrial applications due to their durability and high power output. Long-haul trucks powered by fuel cells offer extended driving ranges and quick refueling, addressing the limitations of battery-powered alternatives. Companies like Nikola and Hyundai have developed hydrogen-powered trucks capable of competing with diesel engines in terms of performance and efficiency.
Industrial applications also leverage the modular design of fuel cell stacks. Forklifts, cranes, and other machinery benefit from the clean energy provided by hydrogen fuel cells. These machines operate efficiently in warehouses and construction sites, where emissions and noise reduction are critical. The ability of fuel cell stacks to withstand harsh conditions ensures their reliability in demanding environments.
“Fuel cells have quickly emerged as a means to enable long-haul trucking, with the potential to surpass the market share of diesel-powered trucks.”
Case Studies by Ningbo VET Energy Technology Co.
Ningbo VET Energy Technology Co. has played a transformative role in advancing fuel cell stack applications. The company has collaborated with global partners to integrate its innovative fuel cell stacks into various real-world projects. For instance, its fuel cell systems have been successfully deployed in public buses across Asia, demonstrating exceptional performance and reliability.
In the industrial sector, Ningbo VET Energy Technology Co. has supplied fuel cell stacks for forklifts and heavy machinery. These applications highlight the adaptability of their technology in meeting diverse energy needs. By focusing on durability and efficiency, the company ensures that its products deliver consistent results even under challenging conditions.
“Ningbo VET Energy Technology Co. continues to drive innovation in fuel cell technology, enabling sustainable solutions across multiple industries.”
Fuel cell stacks have proven their versatility and effectiveness in real-world applications. From passenger cars to industrial machinery, they provide a sustainable energy solution that meets the demands of modern transportation and industry. Their ability to combine efficiency, durability, and environmental benefits positions them as a cornerstone of the future energy landscape.
Fuel cell stacks represent a transformative leap in electric vehicle technology. Their unmatched efficiency, zero-emission operation, and rapid refueling capabilities address critical limitations of battery-powered systems. By stacking individual fuel cells, manufacturers achieve scalable designs tailored to diverse power needs, from passenger cars to heavy-duty vehicles. This versatility makes fuel cell stacks an attractive solution for sustainable transportation. Companies like Ningbo VET Energy Technology Co. continue to innovate, driving advancements that enhance durability and performance. As these technologies evolve, fuel cell stacks are poised to redefine the future of mobility, offering cleaner and more efficient energy solutions.
よくあるご質問
What is a fuel cell stack?
A fuel cell stack is the core component of a fuel cell system. It consists of multiple individual fuel cells connected in series or parallel to generate the required power output. Each fuel cell contains an anode, a cathode, and an electrolyte. Hydrogen and oxygen react within the stack to produce electricity, water, and heat. This process powers electric vehicles efficiently and sustainably. Different types of fuel cell stacks, such as PEM (Proton Exchange Membrane) fuel cells, are designed to operate with specific fuels like hydrogen.
How energy efficient are fuel cell stacks?
Fuel cell stacks are highly energy efficient compared to traditional combustion engines. While internal combustion engines typically achieve an efficiency of around 27%, fuel cell stacks can reach efficiency ratings of up to 60% or more. For instance, Horizon’s H-1000 XP Fuel Cell Stack has demonstrated an efficiency of 59% under real-world conditions. This high efficiency ensures that more energy from hydrogen fuel is converted into usable power, making fuel cell stacks a superior choice for sustainable energy solutions.
How long do fuel cell stacks last?
Fuel cell stacks are known for their durability and longevity. Unlike batteries, which degrade over time, fuel cell stacks are designed to withstand harsh conditions and maintain performance over extended periods. Their robust construction ensures they can endure thousands of operational cycles without significant efficiency loss. This durability provides long-term value, making them a reliable investment for both personal and commercial applications.
What are the environmental benefits of fuel cell stacks?
Fuel cell stacks offer significant environmental advantages. They produce zero harmful emissions, emitting only water vapor and warm air as byproducts. This clean energy generation process helps reduce greenhouse gas emissions and air pollution. By replacing internal combustion engines with fuel cell stacks, vehicles contribute to global efforts to combat climate change and achieve carbon neutrality.
How do fuel cell stacks compare to batteries in electric vehicles?
Fuel cell stacks and batteries serve different purposes in electric vehicles. Fuel cell stacks excel in applications requiring long driving ranges and quick refueling. They can refuel in under five minutes and provide ranges exceeding 300 miles. Batteries, on the other hand, are more suitable for short commutes and urban environments due to their established charging infrastructure. Both technologies complement each other, addressing diverse transportation needs.
What type of fuel do fuel cell stacks use?
Most fuel cell stacks, such as PEM fuel cells, operate using hydrogen as their primary fuel source. Hydrogen is stored in high-pressure tanks within the vehicle and reacts with oxygen in the fuel cell stack to generate electricity. Other types of fuel cells, like direct methanol fuel cells (DMFCs), use methanol as fuel. Choosing the right fuel cell stack depends on the intended application and the availability of compatible fuel.
Are fuel cell stacks cost-effective?
Fuel cell stacks may have a higher upfront cost compared to traditional energy systems, but their efficiency and durability make them cost-effective in the long run. Their ability to convert up to 60% of hydrogen’s energy into electricity reduces fuel consumption. Additionally, their long lifespan minimizes replacement costs, providing significant value over time.
Can fuel cell stacks operate in extreme conditions?
Fuel cell stacks are designed to perform reliably in a wide range of environmental conditions. Their robust construction allows them to withstand temperature fluctuations, humidity, and mechanical stress. Advanced cooling mechanisms and durable materials ensure consistent performance even in demanding applications, such as heavy-duty vehicles and industrial machinery.
How quickly can a fuel cell vehicle refuel?
Fuel cell vehicles refuel much faster than battery-powered electric vehicles. Hydrogen tanks can be fully refueled in under five minutes, similar to the refueling time of traditional gasoline vehicles. This quick refueling capability makes fuel cell vehicles ideal for long-distance travel and commercial operations where downtime must be minimized.
What role does Ningbo VET Energy Technology Co. play in fuel cell innovation?
Ningbo VET Energy Technology Co. is a leader in advancing fuel cell stack technology. The company focuses on enhancing efficiency, durability, and scalability through innovative designs and materials. By improving key components like the membrane electrode assembly (MEA) and integrating advanced cooling systems, Ningbo VET Energy Technology Co. ensures reliable and high-performance fuel cell stacks. Their contributions drive the adoption of fuel cell technology across various industries and applications.