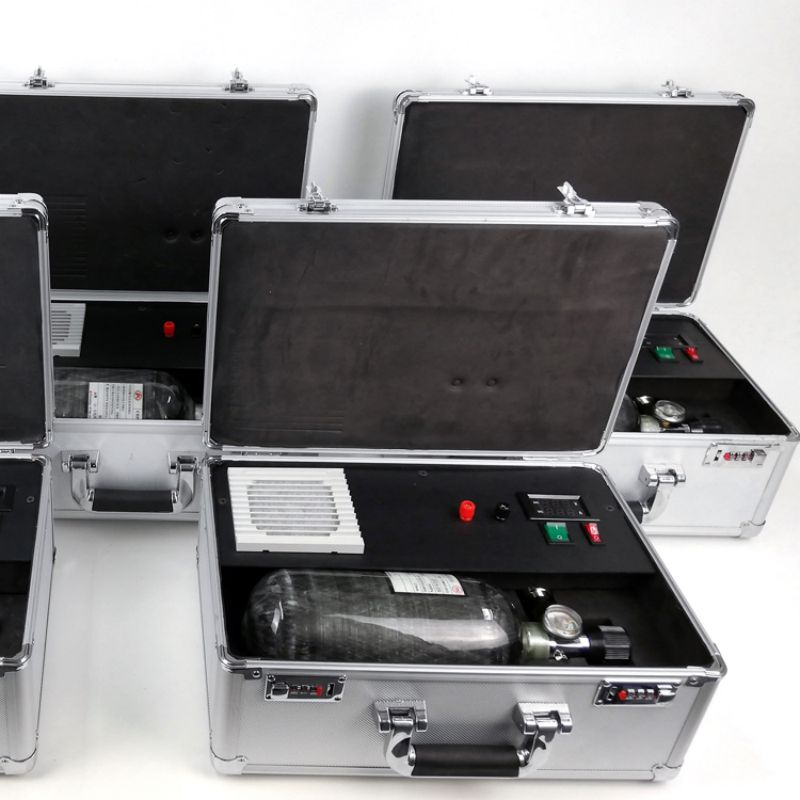
A fuel cell system transforms chemical energy into electricity through an electrochemical reaction. Unlike traditional combustion engines, it operates with remarkable efficiency and minimal environmental impact. Hydrogen serves as the primary fuel, combining with oxygen to produce electricity, water, and heat as byproducts. This clean energy solution offers efficiencies exceeding 60% in combined heat and power systems, surpassing conventional technologies like coal plants. Its quiet operation and zero-emission output make it ideal for diverse applications, including powering innovative technologies like the fuel cell for UAV, which demands reliability and sustainability.
要点
- Fuel cell systems convert chemical energy into electricity through an electrochemical reaction, offering high efficiency and minimal environmental impact.
- Hydrogen and oxygen are the primary reactants, producing only water and heat as byproducts, making fuel cells a clean energy solution.
- Key components of a fuel cell system include the fuel cell stack, hydrogen storage system, oxygen supply system, and power conditioning unit, all working together for optimal performance.
- Fuel cells are versatile and scalable, suitable for various applications such as transportation, stationary power generation, and portable power solutions.
- The environmental benefits of fuel cells include zero emissions and the potential for using renewable hydrogen, aligning with global sustainability goals.
- Challenges such as high costs and hydrogen production/storage need to be addressed for wider adoption, but ongoing innovations are paving the way for future advancements.
- Companies like Ningbo VET Energy Technology Co. are crucial in driving fuel cell technology forward, enhancing efficiency and reliability across multiple sectors.
How Does a Fuel Cell System Work?
The Role of Hydrogen and Oxygen
Hydrogen and oxygen play essential roles in the operation of a fuel cell system. Hydrogen, often stored in pressurized containers, serves as the primary fuel. Oxygen, sourced from the surrounding air, acts as the oxidant. Together, these elements drive the electrochemical reaction that generates electricity. Unlike combustion-based systems, this process does not burn hydrogen. Instead, it uses the chemical energy stored in hydrogen molecules to produce power cleanly and efficiently. This unique approach ensures that the only byproducts are water and heat, making the system environmentally friendly.
The Electrochemical Reaction Process
The core of a fuel cell system lies in its electrochemical reaction. This process begins at the anode, where hydrogen molecules split into protons and electrons. The protons pass through a specialized electrolyte membrane, while the electrons travel through an external circuit, creating an electric current. At the cathode, oxygen combines with the protons and electrons to form water. This continuous flow of electrons through the external circuit generates usable electricity. The absence of combustion eliminates harmful emissions, setting fuel cells apart as a sustainable energy solution.
Outputs: Electricity, Water, and Heat
A fuel cell system produces three primary outputs: electricity, water, and heat. The electricity powers various applications, from vehicles to stationary systems. The water, formed during the reaction, is pure and can be safely released into the environment. Heat, another byproduct, can be captured and utilized in combined heat and power (CHP) systems to improve overall efficiency. These outputs highlight the versatility and sustainability of fuel cell systems, making them a promising alternative to traditional energy sources.
Key Components of a Fuel Cell System
Fuel Cell Stack
について fuel cell stack serves as the core of a fuel cell system. It generates electricity by combining individual fuel cells in series to achieve the required voltage and power output. Each fuel cell within the stack consists of three main components: an anode, a cathode, and an electrolyte membrane. At the anode, hydrogen molecules split into protons and electrons. The protons pass through the electrolyte membrane, while the electrons travel through an external circuit, creating an electric current. At the cathode, oxygen combines with the protons and electrons to form water.
A single fuel cell typically produces between 0.5 and 0.8 volts, which is insufficient for most applications. To overcome this limitation, hundreds of fuel cells are stacked together. This arrangement not only increases voltage but also enhances the system’s overall power output. The size of each cell and the operating conditions, such as temperature and gas pressure, significantly influence the stack’s performance. For example, larger cells provide more reaction sites, enabling higher current generation. This scalability allows fuel cell stacks to power a wide range of applications, from vehicles to stationary energy systems.
Hydrogen Storage System
The hydrogen storage system ensures a consistent supply of hydrogen to the fuel cell stack. Hydrogen, the primary fuel for the system, is typically stored in pressurized tanks. These tanks are designed to withstand high pressures, ensuring safety and efficiency. Advanced materials, such as carbon fiber composites, are often used to construct these tanks, making them lightweight yet durable.
Hydrogen storage systems play a critical role in determining the range and operational efficiency of fuel cell-powered devices. For instance, in vehicles, the storage capacity directly impacts the driving range. Innovations in hydrogen storage technology, such as metal hydrides and cryogenic storage, aim to increase capacity while reducing weight and size. These advancements are essential for expanding the adoption of fuel cell systems in various sectors.
Oxygen Supply System
The oxygen supply system delivers oxygen to the cathode of the fuel cell stack. Unlike hydrogen, oxygen is not stored but sourced directly from the surrounding air. Compressors or blowers are used to draw in air and regulate its flow and pressure. This ensures optimal conditions for the electrochemical reaction within the fuel cell.
The efficiency of the oxygen supply system significantly affects the overall performance of the fuel cell system. Proper airflow management minimizes energy losses and maximizes power output. Additionally, filters are often incorporated to remove impurities from the air, protecting the fuel cell stack from potential damage. By maintaining a steady and clean oxygen supply, this system ensures the reliability and longevity of the fuel cell system.
Power Conditioning Unit
について power conditioning unit (PCU) plays a vital role in ensuring the usability of the electricity generated by the fuel cell system. Fuel cells produce direct current (DC) electricity, which is not compatible with most modern electrical devices and systems that require alternating current (AC). The PCU bridges this gap by converting DC into AC, enabling the electricity to power a wide range of applications, including residential, industrial, and transportation systems.
In addition to converting current, the PCU regulates voltage and frequency to maintain a stable power supply. This regulation ensures that the electricity meets the specific requirements of the connected devices, preventing potential damage caused by fluctuations. Advanced PCUs also incorporate safety features, such as overload protection and fault detection, to enhance the reliability of the fuel cell system.
Modern PCUs are designed to operate efficiently, minimizing energy losses during the conversion process. This efficiency contributes to the overall performance of the fuel cell system, making it a more sustainable energy solution. For instance, in electric vehicles powered by fuel cells, the PCU ensures smooth operation by delivering consistent power to the motor and other onboard systems.
Cooling and Water Management Systems
Effective cooling and water management systems are essential for maintaining the optimal performance of a fuel cell system. During operation, the electrochemical reaction generates heat and water as byproducts. Without proper management, excessive heat can damage the fuel cell stack, while water accumulation can disrupt the reaction process.
The cooling system dissipates heat generated within the fuel cell stack, preventing overheating. It typically uses liquid or air-based cooling methods, depending on the size and application of the system. For example, larger stationary fuel cell systems often rely on liquid cooling due to its higher efficiency, while smaller portable systems may use air cooling for simplicity and cost-effectiveness.
Water management systems ensure that the water produced during the reaction does not interfere with the fuel cell’s operation. These systems remove excess water from the fuel cell stack while maintaining adequate hydration of the electrolyte membrane. Proper membrane hydration is crucial for sustaining the electrochemical reaction and maximizing the system’s efficiency.
Advanced cooling and water management technologies enhance the durability and reliability of fuel cell systems. By maintaining stable operating conditions, these systems extend the lifespan of the fuel cell stack and improve its overall performance. For instance, in combined heat and power (CHP) applications, the heat captured by the cooling system can be repurposed for heating buildings or industrial processes, further increasing the system’s efficiency.
Types of Fuel Cells
Fuel cells come in various types, each designed to meet specific energy needs and applications. These types differ in their operating principles, materials, and temperature ranges. Understanding these distinctions helps in selecting the right fuel cell for a particular purpose.
Proton Exchange Membrane Fuel Cells (PEMFC)
Proton Exchange Membrane Fuel Cells (PEMFC) operate at relatively low temperatures, typically between 50°C and 100°C. They use a solid polymer electrolyte to conduct protons from the anode to the cathode. Hydrogen serves as the fuel, while oxygen from the air acts as the oxidant. The electrochemical reaction in PEMFCs generates electricity, water, and heat.
PEMFCs are known for their quick start-up times and high power density. These features make them ideal for applications such as transportation, including fuel cell electric vehicles (FCEVs). Their compact design also suits portable power systems. However, the need for high-purity hydrogen and precise water management systems adds complexity to their operation. Advanced cooling systems, like those used in Hydrogen Fuel Cell Cooling Systems, ensure efficient heat dissipation, preventing overheating and maintaining optimal performance.
Solid Oxide Fuel Cells (SOFC)
Solid Oxide Fuel Cells (SOFC) operate at high temperatures, ranging from 600°C to 1,000°C. They use a solid ceramic electrolyte to conduct oxygen ions from the cathode to the anode. Unlike PEMFCs, SOFCs can utilize a variety of fuels, including hydrogen, natural gas, and biogas. This flexibility makes them suitable for stationary power generation and industrial applications.
The high operating temperature of SOFCs allows for internal fuel reforming, eliminating the need for external reformers. This increases efficiency but requires robust materials to withstand thermal stress. The heat generated can be repurposed in combined heat and power (CHP) systems, enhancing overall energy efficiency. For instance, in industrial settings, the heat can support processes like steam generation, reducing energy waste.
Alkaline Fuel Cells (AFC)
Alkaline Fuel Cells (AFC) use an alkaline electrolyte, such as potassium hydroxide, to conduct hydroxide ions from the cathode to the anode. These fuel cells operate at temperatures between 60°C and 90°C. Hydrogen and oxygen serve as the primary reactants, producing electricity, water, and heat as byproducts.
AFCs are among the oldest types of fuel cells and have been used in space missions due to their high efficiency and reliability. They perform well with pure hydrogen and oxygen but are sensitive to impurities, which can reduce their lifespan. Despite this limitation, AFCs remain a viable option for specialized applications requiring high efficiency and low operating temperatures.
Molten Carbonate Fuel Cells (MCFC)
Molten Carbonate Fuel Cells (MCFC) operate at high temperatures, typically around 600°C to 700°C. These fuel cells use a molten carbonate salt mixture as the electrolyte, which conducts carbonate ions from the cathode to the anode. Hydrogen, natural gas, or biogas can serve as the fuel, while oxygen or air acts as the oxidant. This flexibility in fuel choice makes MCFCs suitable for large-scale stationary power generation.
MCFCs excel in efficiency, often reaching levels of 50% to 60% for electricity generation alone. When combined heat and power (CHP) systems capture the heat byproduct, overall efficiency can exceed 80%. This makes them ideal for industrial applications where both electricity and heat are required. For example, factories and chemical plants can use MCFCs to power operations while utilizing the heat for processes like steam generation.
The high operating temperature of MCFCs eliminates the need for expensive catalysts, such as platinum, which are required in other fuel cell types. Instead, they use more affordable materials, reducing costs. However, this temperature also demands robust materials to withstand thermal stress and corrosion. Advanced cooling systems, like Hydrogen Fuel Cell Cooling Systems, play a critical role in maintaining stable operation by efficiently dissipating heat and preventing overheating.
MCFCs also contribute to environmental sustainability. They can utilize carbon dioxide from external sources, such as industrial emissions, in their operation. This capability not only reduces greenhouse gas emissions but also aligns with carbon capture and utilization (CCU) strategies. By integrating MCFCs into energy systems, industries can achieve cleaner energy production while addressing environmental challenges.
Phosphoric Acid Fuel Cells (PAFC)
Phosphoric Acid Fuel Cells (PAFC) use liquid phosphoric acid as the electrolyte to conduct hydrogen ions from the anode to the cathode. These fuel cells operate at moderate temperatures, typically between 150°C and 200°C. Hydrogen serves as the primary fuel, while oxygen from the air acts as the oxidant. PAFCs are among the most established fuel cell technologies, with decades of proven performance in stationary power applications.
PAFCs deliver reliable and efficient power, achieving electrical efficiencies of around 40% to 50%. When integrated into CHP systems, overall efficiency can rise to 80%. This makes them well-suited for applications such as hospitals, hotels, and office buildings, where consistent power and heat are essential. For instance, a hospital can use a PAFC system to generate electricity for medical equipment while using the heat to maintain indoor temperatures.
The durability of PAFCs stands out as a key advantage. These fuel cells can tolerate impurities in the hydrogen fuel, such as carbon monoxide, better than many other types. This tolerance simplifies fuel processing and reduces operational costs. Additionally, the moderate operating temperature minimizes material degradation, extending the lifespan of the system.
To ensure optimal performance, PAFC systems rely on auxiliary components like compressors, pumps, and control units. These components regulate the supply of hydrogen, air, and coolant to the fuel cell stack. Products like Fuel Cell System Components integrate these elements seamlessly, enabling safe and efficient operation. Advanced cooling technologies also play a role in maintaining stable temperatures, ensuring the longevity and reliability of the system.
PAFCs contribute to environmental goals by producing minimal emissions. The only byproducts are water and heat, making them a cleaner alternative to traditional fossil fuel-based power generation. Their proven track record and adaptability position them as a valuable solution for sustainable energy needs in both commercial and industrial settings.
Applications of Fuel Cell Systems
Fuel cell systems have revolutionized energy solutions across various sectors. Their versatility, efficiency, and environmental benefits make them suitable for diverse applications. Below are the primary areas where fuel cell systems are making a significant impact.
Transportation
Fuel cell systems are transforming the transportation industry by providing clean and efficient power for vehicles. Hydrogen fuel cell electric vehicles (FCEVs) use Proton Exchange Membrane Fuel Cells (PEMFC) due to their compact design, lightweight structure, and high power density. These vehicles emit only water vapor, making them an eco-friendly alternative to traditional internal combustion engines.
Public transportation systems, such as buses and trains, are increasingly adopting fuel cell technology. For instance, hydrogen-powered buses operate quietly and produce zero emissions, improving air quality in urban areas. Additionally, fuel cell systems are being integrated into maritime vessels and aircraft, including unmanned aerial vehicles (UAVs), where reliability and sustainability are critical.
The long driving range and quick refueling times of hydrogen-powered vehicles address limitations faced by battery electric vehicles (BEVs). For example, a hydrogen-powered car can refuel in minutes and travel hundreds of miles, making it ideal for long-distance travel. This advantage positions fuel cell systems as a key player in the future of sustainable transportation.
Stationary Power Generation
Fuel cell systems excel in stationary power generation, offering reliable and efficient energy for residential, commercial, and industrial applications. Solid Oxide Fuel Cells (SOFC) are particularly well-suited for this purpose due to their high efficiency and ability to operate continuously. These systems can utilize various fuels, including natural gas and biogas, making them versatile for different energy needs.
Combined heat and power (CHP) systems often incorporate fuel cells to maximize energy efficiency. For instance, a factory using an SOFC-based CHP system can generate electricity while capturing the heat byproduct for industrial processes. This dual functionality reduces energy waste and lowers operational costs.
Hospitals, data centers, and other facilities requiring uninterrupted power supply benefit from the reliability of fuel cell systems. Unlike traditional generators, fuel cells operate quietly and produce minimal emissions, ensuring a cleaner and more sustainable energy source. Their scalability also allows customization to meet specific power demands, from small residential units to large industrial setups.
Portable Power
Portable power applications highlight the adaptability of fuel cell systems. These systems provide lightweight and efficient energy solutions for devices and equipment used in remote or off-grid locations. For example, portable fuel cells power camping gear, military equipment, and emergency response tools, ensuring consistent energy supply in challenging environments.
The compact design and high energy density of Proton Exchange Membrane Fuel Cells (PEMFC) make them ideal for portable applications. Unlike traditional batteries, fuel cells do not require recharging. Instead, they continue to operate as long as fuel is supplied, offering a practical solution for extended use.
In disaster relief scenarios, portable fuel cell systems provide critical power for communication devices, medical equipment, and lighting. Their ability to function without relying on grid infrastructure makes them invaluable during emergencies. Additionally, their environmentally friendly operation aligns with global efforts to reduce carbon footprints, even in temporary setups.
Advantages of Fuel Cell Systems
環境面でのメリット
Fuel cell systems offer significant environmental advantages by producing clean energy. Unlike traditional combustion-based technologies, they generate electricity through an electrochemical reaction, which eliminates harmful emissions. The only byproducts are water and heat, making them a zero-emission energy source. This feature aligns with global efforts to reduce greenhouse gas emissions and combat climate change.
The use of hydrogen as a fuel further enhances the environmental benefits. Hydrogen can be produced from renewable sources such as water through electrolysis powered by solar or wind energy. This process creates a sustainable energy cycle, reducing dependence on fossil fuels. Additionally, certain types of fuel cells, like Molten Carbonate Fuel Cells (MCFCs), can utilize carbon dioxide from external sources during operation. This capability supports carbon capture and utilization strategies, contributing to a cleaner environment.
High Efficiency
Fuel cell systems achieve remarkable efficiency levels compared to conventional energy technologies. They convert chemical energy directly into electrical energy, bypassing the limitations of thermal energy conversion. This direct process minimizes energy losses, resulting in efficiencies that often exceed 60% for electricity generation alone. When integrated into combined heat and power (CHP) systems, overall efficiency can surpass 80%.
The internal reforming capability of MCFCs exemplifies this efficiency. These fuel cells convert energy-dense fuels like natural gas and biogas into hydrogen within the cell itself. This eliminates the need for external reformers, reducing energy consumption and operational costs. The high operating temperatures of MCFCs also enable the repurposing of heat byproducts, further enhancing energy utilization.
Versatility and Scalability
Fuel cell systems demonstrate exceptional versatility and scalability, making them suitable for a wide range of applications. Their modular design allows for customization to meet specific energy demands, from small portable devices to large industrial setups. For instance, Proton Exchange Membrane Fuel Cells (PEMFCs) are ideal for transportation due to their compact size and high power density, while Solid Oxide Fuel Cells (SOFCs) excel in stationary power generation with their ability to use various fuels.
The scalability of fuel cell systems extends to their integration into existing energy infrastructures. They can complement renewable energy sources by providing reliable backup power during periods of low solar or wind activity. In industrial settings, fuel cells can support processes requiring both electricity and heat, optimizing resource utilization. This adaptability positions fuel cell systems as a cornerstone of future energy solutions.
Challenges Facing Fuel Cell Systems
High Costs
Fuel cell systems face significant cost challenges, primarily due to the materials and manufacturing processes involved. The fuel cell stack, which serves as the core of the system, often relies on expensive components like platinum-based catalysts. These catalysts enhance the electrochemical reaction but contribute substantially to the overall cost. Additionally, the production of hydrogen, the primary fuel, requires advanced technologies such as electrolysis or steam methane reforming, which further increases expenses.
Scaling up production could help reduce costs. Historically, technologies like solar panels and lithium-ion batteries experienced similar cost barriers during their early stages. Over time, mass production and technological advancements lowered their prices, making them more accessible. A similar trajectory is expected for fuel cell systems as demand grows and manufacturing processes improve. For example, modular designs, which allow systems to be tailored to specific energy needs, could streamline production and reduce material waste.
Efforts to develop alternative materials also aim to address cost concerns. Researchers are exploring non-precious metal catalysts and innovative designs that require fewer raw materials. These advancements could make fuel cell systems more affordable for widespread adoption across industries.
Hydrogen Production and Storage
Hydrogen production and storage present another critical challenge for fuel cell systems. While hydrogen is the most abundant element in the universe, isolating it in its pure form requires energy-intensive processes. Electrolysis, which splits water into hydrogen and oxygen, demands significant electricity, often sourced from fossil fuels. This reliance on non-renewable energy undermines the environmental benefits of fuel cell systems.
Storage adds another layer of complexity. Hydrogen must be stored under high pressure or at extremely low temperatures to ensure safety and efficiency. Pressurized tanks, commonly used for storage, require advanced materials like carbon fiber composites to withstand the stress. These materials increase costs and limit the scalability of hydrogen storage solutions.
Innovations in hydrogen production and storage are addressing these issues. Renewable energy sources, such as wind and solar power, are increasingly being used to produce green hydrogen through electrolysis. This approach reduces the carbon footprint of hydrogen production. Additionally, emerging storage technologies, such as metal hydrides and cryogenic systems, offer promising alternatives. These advancements aim to make hydrogen more accessible and practical for use in fuel cell systems.
耐久性と寿命
The durability and longevity of fuel cell systems remain key concerns, particularly for applications requiring consistent performance over extended periods. The fuel cell stack, exposed to high temperatures and reactive gases, experiences wear and tear over time. Degradation of components, such as the electrolyte membrane and catalysts, reduces efficiency and shortens the system’s lifespan.
Environmental factors also impact durability. Impurities in hydrogen or oxygen can poison the catalysts, leading to performance losses. For instance, alkaline fuel cells, which have been used in space missions since the 1960s, require pure hydrogen and oxygen to maintain reliability. This sensitivity to impurities limits their use in broader applications.
To enhance durability, researchers are developing more robust materials and designs. Advanced cooling and water management systems help maintain stable operating conditions, reducing thermal and mechanical stress on the fuel cell stack. Innovations in catalyst technology, such as the use of nanostructured materials, aim to improve resistance to degradation. These efforts are critical for ensuring the long-term viability of fuel cell systems in diverse applications, from transportation to stationary power generation.
The Future of Fuel Cell Technology
Innovations in Materials and Design
Advancements in materials and design are driving the evolution of fuel cell technology. Researchers are focusing on developing more efficient and durable components to enhance performance. For instance, non-precious metal catalysts are emerging as alternatives to platinum, significantly reducing costs while maintaining efficiency. These innovations aim to make fuel cells more accessible for widespread adoption.
Design improvements also play a crucial role. Engineers are creating compact and modular fuel cell systems that can be tailored to specific applications. This flexibility allows industries to integrate fuel cells into various energy infrastructures seamlessly. For example, portable fuel cells now feature lightweight designs, making them ideal for off-grid power solutions. These advancements ensure that fuel cell systems remain versatile and scalable, meeting the growing demand for clean energy.
Expansion of Hydrogen Infrastructure
The expansion of hydrogen infrastructure is essential for the future of fuel cell technology. Hydrogen serves as the primary fuel for most fuel cell systems, yet its production, storage, and distribution require significant investment. Governments and private sectors worldwide are collaborating to establish hydrogen refueling stations and production facilities. These efforts aim to create a robust supply chain that supports the adoption of hydrogen-powered technologies.
Renewable energy sources are increasingly being used to produce green hydrogen through electrolysis. This method reduces the carbon footprint of hydrogen production, aligning with global sustainability goals. Countries like Japan and Germany are leading the way by investing heavily in hydrogen infrastructure. Their initiatives include building hydrogen corridors and integrating hydrogen into public transportation systems. These developments pave the way for a cleaner and more sustainable energy future.
Role of Companies like Ningbo VET Energy Technology Co.
Companies like Ningbo VET Energy Technology Co. are at the forefront of advancing fuel cell technology. Their expertise in developing innovative solutions contributes significantly to the industry’s growth. By focusing on research and development, they create high-performance components that enhance the efficiency and reliability of fuel cell systems.
Ningbo VET Energy Technology Co. also plays a vital role in promoting the adoption of fuel cells across various sectors. Their products cater to diverse applications, from transportation to stationary power generation. For instance, their cutting-edge fuel cell stacks and cooling systems ensure optimal performance in challenging environments. These contributions highlight the company’s commitment to driving progress in clean energy technologies.
“The future of fuel cell technology depends on collaboration between innovators, governments, and industries. Companies like Ningbo VET Energy Technology Co. exemplify the potential of such partnerships to transform global energy systems.”
Potential for Global Energy Transition
Fuel cell systems hold immense potential to revolutionize the global energy landscape. Their ability to generate clean power with high efficiency positions them as a cornerstone for sustainable energy solutions. Unlike traditional combustion-based technologies, fuel cells convert chemical energy directly into electrical energy, achieving efficiencies that often exceed 60%. This surpasses the efficiency of fossil fuel power plants, which typically operate at around 35%, and internal combustion engines, which achieve only 15% to 25% 効率.
The adoption of fuel cell technology can significantly reduce greenhouse gas emissions. By utilizing hydrogen as a primary fuel, fuel cells produce electricity with water and heat as the only byproducts. This zero-emission process aligns with global efforts to combat climate change and transition to cleaner energy sources. Additionally, integrating biogas into fuel cell systems further enhances sustainability by reducing landfill waste and avoiding combustion-related pollutants.
Governments and industries worldwide are recognizing the transformative potential of fuel cells. Many countries are investing in hydrogen infrastructure to support the widespread adoption of fuel cell systems. For example:
- Japan has established hydrogen corridors and integrated fuel cells into public transportation systems.
- Germany is leading in renewable hydrogen production, using solar and wind energy to create green hydrogen through electrolysis.
- The United States is advancing hydrogen refueling networks to promote fuel cell electric vehicles (FCEVs).
These initiatives demonstrate a collective commitment to reducing reliance on fossil fuels and fostering a cleaner energy future.
Fuel cells also offer versatility across various applications, from transportation to stationary power generation. In the transportation sector, hydrogen-powered vehicles provide long driving ranges and quick refueling times, addressing limitations faced by battery electric vehicles. For stationary power, fuel cells deliver reliable energy for hospitals, data centers, and industrial facilities, ensuring uninterrupted operations while minimizing environmental impact.
The scalability of fuel cell systems further supports their role in the global energy transition. Modular designs allow for customization to meet diverse energy demands, from small portable devices to large-scale industrial setups. This adaptability ensures that fuel cells can integrate seamlessly into existing energy infrastructures, complementing renewable energy sources like solar and wind.
“Fuel cells represent a pivotal step toward a sustainable energy future. Their high efficiency, environmental benefits, and versatility make them a key player in the global shift away from fossil fuels.”
As advancements in materials, design, and hydrogen infrastructure continue, fuel cell systems will play an increasingly vital role in transforming energy systems worldwide. Their potential to drive the global energy transition underscores their importance in achieving a cleaner, more sustainable future.
Fuel cell systems represent a transformative step toward sustainable energy solutions. Their ability to deliver high efficiency, zero emissions, and reliable performance positions them as a superior alternative to traditional combustion engines and batteries. Unlike batteries, fuel cells do not degrade over time and offer faster refueling, making them practical for diverse applications. Companies like Ningbo VET Energy Technology Co. continue to innovate, driving advancements that address current challenges. With ongoing technological progress and expanding hydrogen infrastructure, fuel cell systems hold immense potential to revolutionize global energy systems and support a cleaner future.
よくあるご質問
What is a fuel cell, and how does it work?
A fuel cell generates electricity through an electrochemical reaction rather than combustion. It combines hydrogen and oxygen to produce electricity, water, and heat. The process begins at the anode, where hydrogen molecules split into protons and electrons. The protons pass through an electrolyte membrane, while the electrons create an electric current by flowing through an external circuit. At the cathode, oxygen reacts with the protons and electrons to form water.
What are the main components of a fuel cell system?
A fuel cell system consists of several key components:
- Fuel Cell Stack: The core unit where the electrochemical reaction occurs.
- Hydrogen Storage System: Stores hydrogen fuel in pressurized tanks.
- Oxygen Supply System: Sources oxygen from the air for the reaction.
- Power Conditioning Unit (PCU): Converts direct current (DC) electricity into alternating current (AC).
- Cooling and Water Management Systems: Regulate temperature and manage water byproducts.
These components work together to ensure efficient and reliable energy production.
What types of fuel cells are available?
Fuel cells come in various types, each suited for specific applications:
- Proton Exchange Membrane Fuel Cells (PEMFC): Ideal for transportation and portable power.
- Solid Oxide Fuel Cells (SOFC): Suitable for stationary power generation.
- Alkaline Fuel Cells (AFC): Used in specialized applications like space missions.
- Molten Carbonate Fuel Cells (MCFC): Designed for industrial and large-scale power needs.
- Phosphoric Acid Fuel Cells (PAFC): Commonly used in commercial and residential settings.
Each type varies in operating temperature, materials, and efficiency.
What are the advantages of fuel cell systems?
Fuel cell systems offer several benefits:
- 環境面でのメリット: Zero emissions, producing only water and heat as byproducts.
- High Efficiency: Converts chemical energy directly into electricity with minimal losses.
- 汎用性: Powers a wide range of applications, from vehicles to industrial systems.
- Quiet Operation: Produces energy without noise, making it suitable for urban and residential areas.
These advantages make fuel cells a promising clean energy solution.
How is hydrogen stored for fuel cell systems?
Hydrogen is typically stored in pressurized tanks made from advanced materials like carbon fiber composites. These tanks ensure safety and efficiency by withstanding high pressures. Emerging technologies, such as metal hydrides and cryogenic storage, aim to increase storage capacity while reducing weight and size. Proper storage solutions are essential for the performance and range of fuel cell-powered devices.
What applications use fuel cell systems?
Fuel cell systems are used in various sectors:
- Transportation: Powers hydrogen fuel cell electric vehicles (FCEVs), buses, trains, and UAVs.
- Stationary Power Generation: Provides reliable energy for homes, hospitals, and factories.
- Portable Power: Supplies energy for camping gear, military equipment, and emergency tools.
Their adaptability and efficiency make them suitable for diverse energy needs.
How does Ningbo VET Energy Technology Co. contribute to fuel cell advancements?
Ningbo VET Energy Technology Co. specializes in developing and manufacturing high-quality fuel cell components. Their product range includes hydrogen fuel cell stacks, membrane electrode assemblies, バイポーラプレートそして PEM electrolyzers. The company focuses on innovation and reliability, ensuring their products meet the demands of modern energy systems. Their contributions support the growth of fuel cell technology across industries.
What makes fuel cells different from batteries?
Fuel cells and batteries both provide electricity, but they operate differently. Fuel cells generate electricity continuously as long as hydrogen and oxygen are supplied. Batteries store energy and require recharging after depletion. Fuel cells also produce higher energy density and faster refueling times, making them ideal for applications requiring long-term and consistent power.
Are fuel cell systems environmentally friendly?
Yes, fuel cell systems are highly environmentally friendly. They produce electricity without harmful emissions, with water and heat as the only byproducts. When powered by green hydrogen—produced using renewable energy sources—they offer a completely sustainable energy cycle. This aligns with global efforts to reduce carbon footprints and combat climate change.
What is the future of fuel cell technology?
The future of fuel cell technology looks promising. Innovations in materials, such as non-precious metal catalysts, aim to reduce costs and improve efficiency. Expanding hydrogen infrastructure will support wider adoption in transportation and power generation. Companies like 寧波VETエネルギー技術有限公司 continue to drive advancements, ensuring fuel cells play a vital role in the global transition to clean energy.